Authors
Shivi Selvaratnam Ph.D., Michael Coughlin Ph.D., Elizabeth Sotkiewicz, Carmel Holliday, Alyssa
Mullens, and Kaila Whited
Abstract
Since ASHRAE 188 (Legionellosis: Risk Management for Building Water Systems) was ratified in 2015, there has been considerable efforts made by Water Management Teams to control the growth of Legionella by commissioning secondary disinfection systems. The methods of secondary disinfection are generally confined to chlorine, monochloramine, chlorine dioxide and copper/silver ionization. Which of these systems is selected will depend upon several factors including water quality, materials of construction and local regulations. The complexity of this decision-making process has increased since the Centers for Medicare and Medicaid Services (CMS) expanded the requirement of a Water Management Plan to control the presence of not only Legionella, but all Opportunistic Waterborne Pathogens. Mycobacteria spp. fall within this category of microorganisms and compared to other Opportunistic Waterborne Pathogens, they are particularly resistant to disinfection due to their comparatively slow respiration and hydrophobic cell wall. Therefore, the decision to select one type of a secondary disinfection system over another should also be based on the ability of that method to curtail the growth of Mycobacteria spp. In this paper we compare the efficacy of traditional secondary disinfection systems to control the growth Legionella and Mycobacterium spp. in potable water systems.
Introduction
The role that opportunistic waterborne pathogens (OWP) play in contributing to hospital acquired infections continues to gain prominence amongst infection control professionals. Pseudomonas aeruginosa has long been known as a water borne pathogen that can cause pneumonia and wound infections. However, it wasn’t until Legionnaires’ Disease caught the attention of the general public and the ubiquity of the causative agent (Legionella spp.) in potable water systems that new guidelines and standards were promulgated. One of the most recent policy decisions developed was that of the Centers for Medicare and Medicaid Services (CMS). A memorandum first issued by CMS in June of 2017 and reissued in July of 2018 to provide clarification, made it clear that health care facilities must reduce the risk of hospital acquired infections caused by any OWP.
Since most testing of OWP has been focused on the control of Legionella spp., there is little information available on the control of other OWP. In this paper we examine the use of secondary disinfectants to control the growth of Legionella spp. , Mycobacterium spp. and HPC. Mycobacterium was selected as an indicator organism because its slow growth and fatty cell wall could make it especially resistant to disinfection. Since the most commonly noted OWP belong to the generic group of bacteria commonly referred to as Heterotrophic Plate Count (HPC) bacteria, we also decided to use this group of bacteria as an indicator of potable water quality. The presence of Pseudomonas spp. was not investigated because in a recent study, it was found to be rarely present and therefore the lack of data would make it impossible to make statistically relevant conclusions.
Materials and Methods
Potable Water Sample Collection
One liter (1 L) potable first draw water sample from faucets of healthcare facilities were collected in sterile wide-mouth screw cap polypropylene plastic bottle containing 150-200 mg sodium thiosulfate preservative. Temperature and disinfectant concentration were recorded two minutes after the faucet was opened at maximum water velocity.
Preparation of Samples for Bacteriological Examination
Filtration of Potable Water Samples for Legionella Isolation
Five hundred ml (500 ml) of each potable water sample was filter-concentrated using a 47-mm filter funnel assembly disinfected with 80% isopropyl alcohol between uses and containing a sterile 0.22 µm polycarbonate filter. After filtration, the filter was removed aseptically from the holder with sterile filter forceps, folded to the outside, and placed into a sterile, 50-ml centrifuge tube containing 5ml of sterile Butterfield’s buffer. The centrifuge tube was then vortexed for one minute at maximum speed to elute bacteria from the filter.
Acid Treatment of Samples for Legionella Isolation
A selective procedure was used to reduce the numbers of non-Legionella bacteria before culturing since water samples may contain high concentrations these bacteria (CDC, 2005). Two hundred (200) µl of the vortexed suspension was placed into a sterile 1.5 ml centrifuge tube containing 200 µl of acid buffer. The suspension was then incubated for 5 minutes at room temperature. For swab samples, 100 µl of the suspension was placed into a sterile 1.5 ml centrifuge tube containing 100 µl of acid buffer and treated as described above.
Media for Legionella Growth and Isolation
Buffered charcoal yeast extract (BCYE) agar containing 0.1% alpha-ketoglutarate was used as the base medium for the recovery of Legionella (1). Two types of selective BCYE agar were used in the processing of the samples. The first was designated BCYE complete with antibiotics (purchased from Hardy Diagnostics); the second, BCYE complete without antibiotics.
Plating of Samples
Plates (described above) were inoculated with 0.2 ml of either acid-treated or non-acid treated suspension and distributed over the agar surface with a plastic spreader. They were then incubated at 36oC in a humidified incubator for 14 days at a minimum humidity of 95%.
Examination of Cultures for Legionella
Plates were examined after 72 to 96 hours of incubation for Legionella. Suspect Legionella colonies were streaked onto BCYE agar plate without L-cysteine and antibiotics, and a positive control BCYE agar plate without antibiotics. The plates were incubated for 24-48 hours. Colonies that grew on BCYE agar, but not BCYE agar without L- cysteine, were considered to be presumptive Legionella species and later serotyped using the Dry Spot ™agglutination test (Oxoid, Dardilly, France) or direct fluorescent antibody (MTech).
Isolation of Nontuberculous Mycobacterium (NTM) from Potable Water
Culturing of NTM was carried out according to Standard Methods for the Examination of Water and Wastewater, Method 9260M using Middlebrook 7H10 agar (Hardy Diagnostics). Twenty ml 0.04% (w/v) cetylpridinium chloride (CPC) was added to 500 ml of water sample and left at room temperature for 24 h. The water sample was then filter-concentrated using a 47-mm filter funnel assembly disinfected with 80% isopropyl alcohol between uses and containing a sterile 0.22 µm polycarbonate filter. After filtration, the filter was removed aseptically from the holder with sterile filter forceps and placed onto a Middlebrook 7H10 agar plate ensuring no air was trapped beneath the membrane. Plates were incubated for 7-14 days at 36°C.
Examination of Cultures of NTM
Plates were examined after 7-14 days of incubation for NTM. Colonies were examined for morphological characteristics typical of NTM. Presumptive NTM colonies were counted and select colonies were stained with acid-fast stain using Ziehl-Neelsen Method.
Results and Discussion
The data in Table 1 indicate that compared to all other treatments, ClO2 is relatively ineffective in controlling HPC counts at levels even below the 0.05 significance level. An examination of the average CFU/ml shows that average CFU/ml is always higher when ClO2 is used to supplement chlorine as compared to all other treatments. With the exception of perhaps Total Chlorine and Copper/Silver, all other treatments performed differently from one another at the 0.05 significance level.
By pairing each of these secondary treatments and conducting a T-test on them, we can compare the effect of potential combination treatments and their ability to control the growth of HPC organisms. Admittedly, these results are obtained in a background of chlorine but it nevertheless may provide guidance for future treatment regimens which may not necessarily include the use of chlorine. Based on the data in Table 1, the combination of monochloramine and copper silver ionization indicates that this could be an effective strategy for controlling the growth of HPC organisms. The use of copper silver ionization is most often reserved for the treatment of hot water systems. Attempts to implement this type of treatment in cold water systems is usually avoided because of staining issues of porcelain materials that come in contact with this disinfectant. Secondary disinfection using monochloramine is also normally associated with the treatment of hot water systems, in part, due to the cost of treating both hot and cold water and the generating capacity of packaged monochloramine systems are generally limited to low water volume systems. Therefore, if a secondary disinfection were to be recommended to control the concentration of HPC organisms below the EPA treatment standard of 500 CFU/ml, one possible solution would be to use copper silver ionization in a potable water system at premise point of entry that is currently being treated with monochloramine. It is unlikely that this scenario would be commonplace as the use of monochloramine delivered by a municipality is itself not a common practice. Chlorine is still the mainstay treatment provided by most municipalities in the US and fortunately, the data in Table 1 also indicates that copper silver ionization coupled with chlorine as the primary disinfectant is also an effective strategy to control HPC bacteria.
Table 1: The Effect of Potential Treatment Combinations on Controlling the Growth of HPC Organisms.
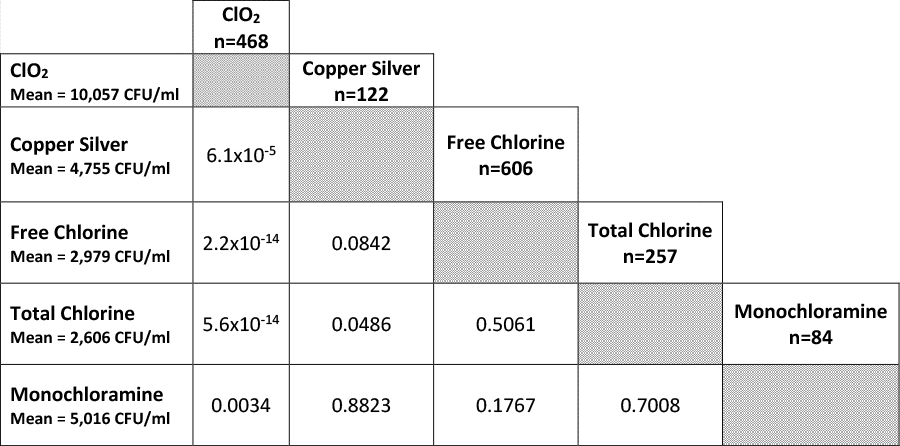
Figure 1: Treatment Means and Standard Deviations for HPC
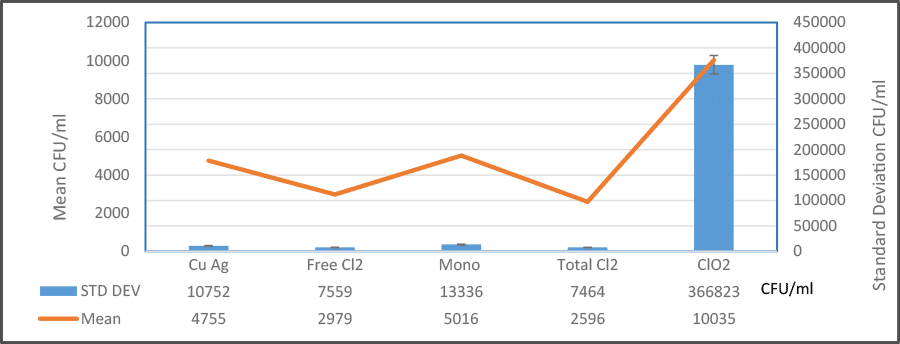
Monochloramine and chlorine dioxide appear to be the preferred disinfectants for controlling the growth of Legionella spp. in the potable water systems that were examined. Free chlorine and total chlorine were significantly less effective when either the percent positivity or the concentration of Legionella spp. were used as indices of performance. The use of copper silver ionization was also associated with a comparatively low rate of positivity but when systems were positive, the concentration of Legionella spp. was surprisingly high. It is not clear why this phenomenon occurs but one possibility is that copper silver ionization is slow to exert its biocidal effect on biofilm that is released from the surface of potable water pipes.
Mycobacterium spp. do indeed appear to be more resilient in their ability to resist the biocidal effects of the tested disinfectants and this may be due to the presence of a hydrophobic cell wall that impedes the penetration of disinfectants and its slow respiration. In the systems that tested positive for Mycobacterium spp., monochloramine and copper silver ionization were associated with the lowest concentration of this OWP. There is no clear explanation for this phenomenon but it may be related to the comparative inertness of these disinfectants to the waxy composition of the cell wall.
Table 2: Percent Positivity and Mean Concentration of Legionella and Mycobacterium
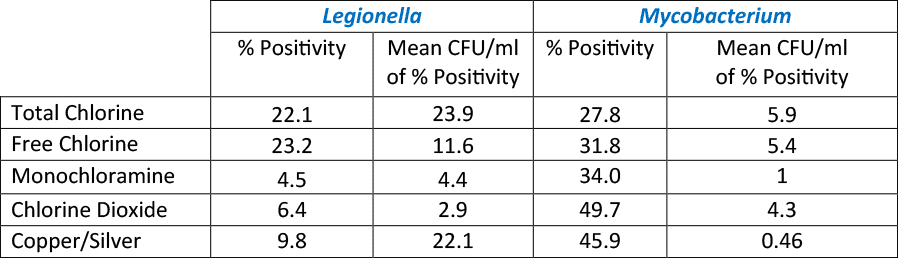
The reason for such high variability does not appear to be correlated with the temperature of the water system. Theoretically, all the tested disinfectants are less stable under higher temperatures. However, the labile nature of the disinfectants does not explain the large range in disinfectant concentration.
Figure 2: Stability of Treatment Concentrations
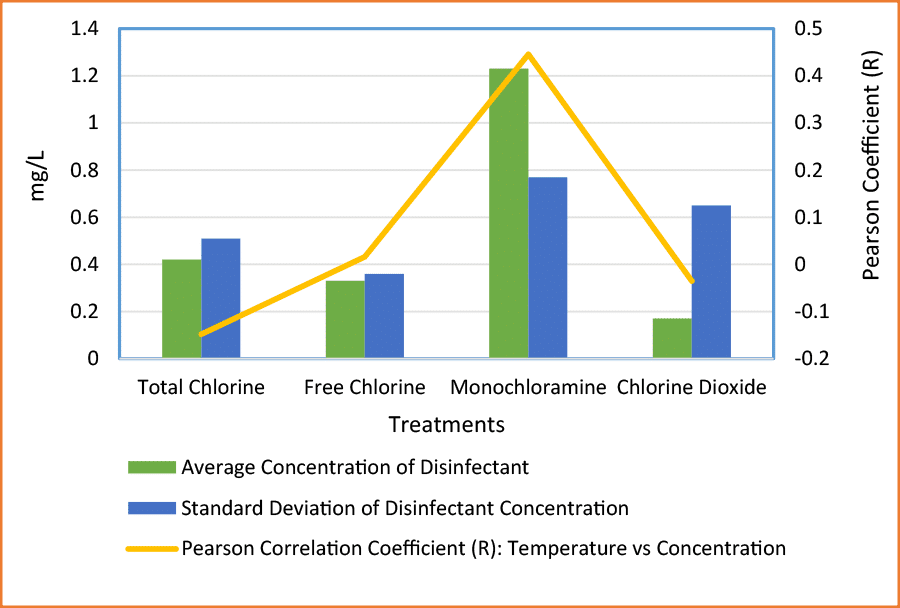
Figure 3: Percent Positive of Total Samples
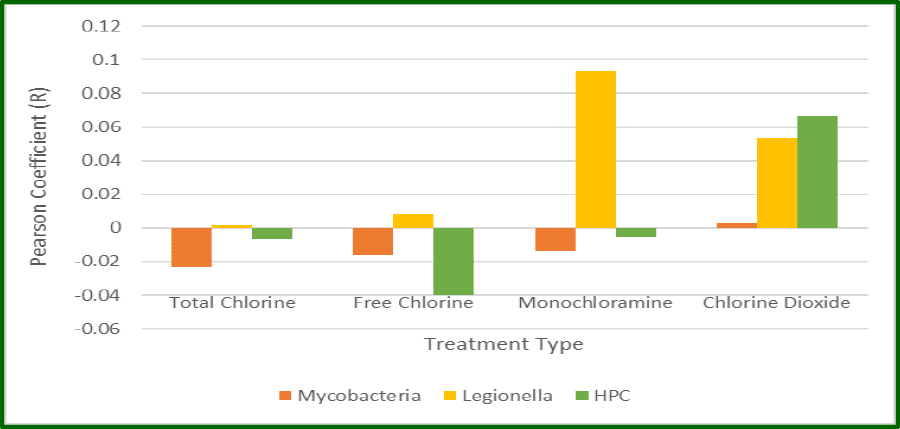
Summary Points
- Keeping Legionella spp. from being present in potable water systems appears to be relatively easy compared to Mycobacterium and HPC with the use of several options including copper silver ionization, monochloramine and chlorine dioxide. In addition to considering the antimicrobial efficacy of a disinfectant against a particular OWP, there are logistical issues that also need to be considered when deciding which disinfectant to use. These factors include the inherent corrosivity of a disinfectant to the materials of construction present in a potable water system, the age of these materials and the cost of installing a disinfectant feed and control system. All of these logistical issues were beyond the scope of this study.
- Good control of the concentration of total chlorine and monochloramine is observed by the median concentrations (1.56 ppm total chlorine, 1.04 ppm monochloramine) and acceptable standard deviations (0.38 ppm total chlorine and 0.32 ppm monochloramine). Therefore, the apparent inability of this combination treatment to control the growth of Mycobacterium and HPC is not due to poor disinfectant control.
- Although Legionella is generally regarded as a chlorine tolerant organism, monochloramine and chlorine dioxide appear to be effective against this OWP. The HPC concentration on the other hand often exceeded the EPA treatment standard of 500 CFU/ml and was difficult to control with any of the tested disinfectants. This is particularly worrisome as virtually all OWP are heterotrophs and the failure of combination treatments (dual and different disinfectants) to control their growth will require strategies that go beyond the use of a supplemental secondary disinfectant.
- Mycobacterium, although present in low concentrations, is prevalent in potable water systems and relatively recalcitrant to conventional disinfection treatments. This phenomenon may be related to the composition of its unique cell wall.